Condensed Matter Physics
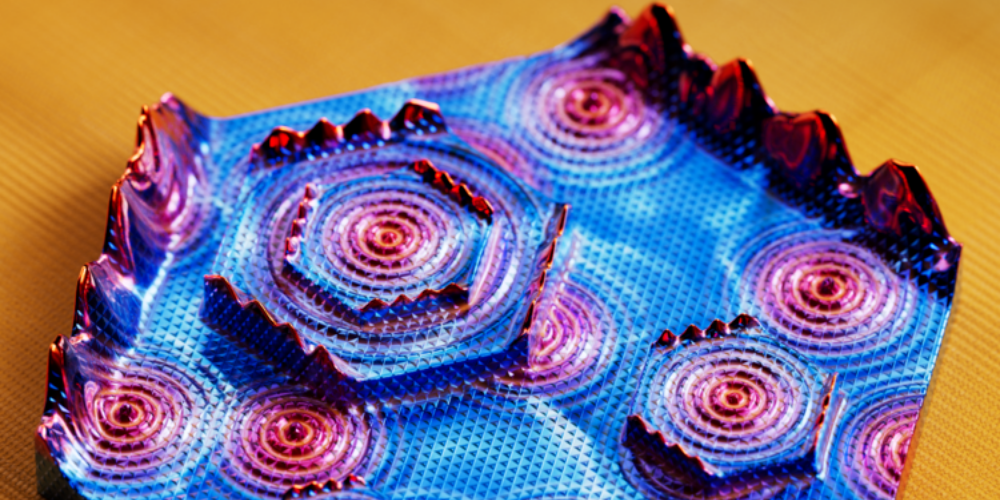
Table of contents
- Prof. Tomáš Bzdušek - Theory of topological matter
- Prof. Titus Neupert - Condensed matter theory
- Prof. Johan Chang - Superconductivity and Magnetism
- Prof. Thomas Greber - Low dimensional systems
- Prof. Marc Janoschek - Correlated Quantum Matter
- Prof. Fabian Natterer - Quantum Matter
- Prof. Johanna Nordlander - Oxide Materials
- Prof. Andreas Schilling - Phase Transitions, Materials and Applications
- PD. Tatiana Latychevskaia (PSI) - Coherent Diffraction Imaging
Prof. Tomáš Bzdušek - Theory of topological matter

Our research focuses on topological phases of classical and quantum matter. Topological matter exhibits properties resilient against a broad range of perturbations, and include phenomena such as quantized Hall conductivity in semiconductor devices or spin-momentum-locked metallic states on the surface of topological insulators.
We use diverse mathematical techniques and numerical approaches to extend the notion of topological invariants to a broader array of physical systems and to study their fingerprints in physical observables, and we propose prospective setups for realizing them in experiments. Besides conventional crystalline solids, we also explore dissipative and periodically driven systems, along with lattices that feature unique curved or aperiodic geometries.
Expander graphs: where boundary scales with volume
Condensed matter research conventionally revolves around systems with crystalline structure. However, recent years have witnessed an increasing interest in non-crystalline matter, including amorphous solids, quasicrystals, and quantum fractals. A yet another intriguing geometry is offered by so-called expander graphs: arrangements of sites where the boundary of any region grows linearly with its volume. Such systems exhibit an emergent negative curvature and prospective connections to holographic principles.
Narrowing attention to regular systems, i.e., ones that look the same from the vantage point of any site, one can distinguish hyperbolic lattices (with closed loops) and Bethe lattices (without closed loops). Our group has recently developed a pair of tandem packages, H YPER C ELLS for GAP and H YPER B LOCH for Mathematica, which enable efficient computation of spectra on hyperbolic lattices. We made documentation and detailed tutorials for these packages available at www.hypercells.net. On the other hand, analytic or iterative solutions for Bethe lattices are readily available. Equipped with these techniques, we set out to study topological phases, the role of correlations, and quantum entanglement in a range of models on expander graphs.
Figure: Phase diagram of the Kitaev spin liquid model on a hyperbolic lattice in applied magnetic field. The two density plots display the energy gap (∆E, left) and the computed Chern number (C, right). By tuning the coupling strengths (Jx,y,z ), one can reach a trivial gapped phase (G) as well as a chiral topological phase (χ) with anyonic excitations and an extensive ground state degeneracy.
Topology and correlations in hyperbolic lattices
Hyperbolic lattices in two spatial dimensions are associated with a higher (four or more) dimensional momentum space. This enables exotic topological phases, including semimetals characterized by higher-dimensional invariants as well as insulators carrying multiple first Chern numbers. Using advanced group-theoretical reasoning, we recently clarified the quantized response associated with the latter scenario. Nevertheless, the community still remains far from a full understanding of topological phases in such curved set-ups.
Highlighted Publications:
- Hyperbolic Non-Abelian Semimetal,
T. Tummuru, A. Chen, P. M. Lenggenhager, T. Neupert, J. Maciejko, and T. Bzdušek,
Phys. Rev. Lett. 132, 206601 (2024) - Topological linear response of hyperbolic Chern insulators,
C. Sun, A. Chen, T. Bzdušek, and J. Maciejko,
SciPost Phys. 17, 124 (2024)
Prof. Titus Neupert - Condensed matter theory
We study topological phases of quantum matter with numerical and analytical tools. Topological electronic states are characterized universal and robust phenomena, such as the Hall conductivity in the integer quantum Hall effect, that are of fundamental interest or promise applications in future electronics. We study and propose concrete materials to realize such topological effects, but are also interested in studying abstract models to understand what phases of matter can exist in principle.
Our numerical toolbox includes neural network algorithms to study strongly interacting quantum many-body systems. Furthermore, we work at the interface of quantum computing and condensed matter physics.
Anomalous Dirac Fermions of topological crystalline insulators
Many concepts in condensed matter physics are in correspondence with high-energy physics. This includes relativistic particle dispersions, Weyl, Dirac and Majorana fermions, or anomalies and topological terms in field theories. However, condensed matter realizations are often not as pristine, plagued by only approximately applicable symmetries and conservation laws, dirty details of experiments and the like.
Very few materials transcend these issues, with graphene being the arguably best known example – realizing 2D massless Dirac fermions to a very good approximation. Among 3D materials, SnTe has similarly spectacular low-energy states. We have studied this material in close collaboration with the experimental group of Matthias Bode and theorists from Ronny Thomale’s group, both at University of Würzburg, in a series of projects over the past years. SnTe is a 3D topological crystalline insulator – a material that is gapped in the bulk, but has protected massless Dirac fermions on the surface.
These Dirac fermions are not spin-degenerate and their massless character is perfectly protected by the topological structure of the system, as long as certain symmetries are not broken. In contrast, graphene’s Dirac cones are actually weakly gapped with a mass set by the small spin-orbit coupling energy scale. The SnTe Dirac fermions have another property – helicity – that sets them apart and is uniquely enabled by the 3D topology of the system. No purely 2D system (that is not attached to a bulk 3D system) could support such states.
- Zoom (PNG, 256 KB)
- Figure: Dirac fermions on the surface of SnTe reveal whether they break chiral (particle-antiparticle) symmetry though their behavior across a topological domain wall on the surface. If chiral symmetry is broken, those with opposite angular momentum (m± ) exchange their energy.
The fundamental laws of physics are dictated by three fundamental symmetries: time-reversal (T), parity (P, space inversion) and charge conjugation (C, particle-antiparticle). However, only their combination CPT is a true symmetry, while C, P, and T are more or less strongly violated individually. Of these, C symmetry is the most mysterious. It also appears as an effective symmetry in condensed matter realizations of the Dirac equation. We have put the Dirac fermions in SnTe to a test and devised a measurement that revealed whether they exhibit chiral symmetry. For that, we exposed the material to a strong magnetic field and measured the surface states with a scanning tunneling microscope. Our analysis revealed that chiral symmetry is subtly broken. This is an instructive example to show how far condensed-matter-to-high-energy analogies can be pushed.
Highlighted Publications:
- Probing chiral symmetry with a topological domain wall sensor,
G. Wagner et al., Newton 1, 100009, (2025);
arXiv:2025.100009. - Interaction Effects in a 1D Flat Band at a Topological Crystalline Step Edge, G. Wagner et al. Nano Letters 23, 2476–2482 (2023).
- Robust spin-polarized midgap states at step edges of topological crystalline insulators,
P. Sessi et al. Science 354, 1269 (2016).
Prof. Johan Chang - Superconductivity and Magnetism
We investigate quantum matter phases emerging from strong electronic interactions. High-temperature superconductivity, strange metals, density-wave instabilities and electronic driven metal insulators metal-insulator transitions are studied by synchrotron techniques. Using angle-resolved photo-emission spectroscopy (ARPES) and resonant inelastic x-ray scattering (RIXS), we reveal electronic structures and properties of such correlated electron systems. Quantum phase transitions tuned by magnetic field, uniaxial or hydrostatic pressure are furthermore explored by high-energy x-ray diffraction. Our group also has technical initiatives to develop innovative and compact cryo-cooling methodology. Finally, we are involved in data science analysing x-ray scattering results using machine learning methodology.
Hallmarks of quantum matter are complex phases emerging from electronic interactions. An experimental progress pathway is to study these phases with advanced spectroscopy techniques. Here, we provide two study examples using electron spectroscopy and resonant x-ray scattering on, respectively, a Mott insulating system and a kagome metal.
Soft Mott Insulator
Quantum fluctuations in low-dimensional systems and near quantum phase transitions have significant influences on material properties. We provide a resonant inelastic x-ray scattering study of magnon excitations in Mott insulating cuprates. From the thin film of SrCuO2 , single- and bi-magnon dispersions are derived. Using an effective Heisenberg Hamiltonian generated from the Hubbard model, we show that the single-magnon dispersion is only described satisfactorily when including significant quantum corrections stemming from magnon-magnon interactions. Comparative re-sults on La2CuO4 indicate that quantum fluctuations are much stronger in SrCuO2 suggesting closer proximity to a magnetic quantum critical point. Our results indicate that SrCuO2 - due to strong quantum fluctuations - is a unique starting point for the exploration of novel magnetic ground states.
- Zoom (PNG, 173 KB)
- Electronic phase diagram of a square lattice with half filling.
Kagome Electronics
Tunable quantum materials hold great potential for applications. The kagome compounds AV3 Sb5 (A = K, Rb, Cs) provide a testbed for electronic tunable states. Through angle-resolved photoemission spectroscopy, we provided the electronic responses induced by compressive and tensile strains on the charge-density-wave (CDW) and van Hove singularity (VHS) in CsV3 Sb5 . We observe a tripling of the CDW gap magnitudes with 1% strain. Simultaneously, changes of both energy and mass of the VHS are observed. Combined, this reveals an anti-correlation between the unconventional CDW order parameter and the mass of the VHS, and highlight the role of the latter in the superconducting pairing.
Highlighted Publications:
- Uniaxial strain tuning of charge modulation and singularity in a kagome superconductor,
C.Lin et al., Nature Communications 15, 10466 (2024) - Magnon interactions in a moderately correlated Mott insulator,
Q. Wang et al., Nature Communications 15, 5348 (2024) - Weak signal extraction enabled by deep neural network denoising of diffraction data,
J. Oppliger et al., Nature Machine Intelligence 6, 180–186 (2024)
Prof. Thomas Greber - Low dimensional systems
We study objects like zero-dimensional endofullerene molecules and two-dimensional (2D) boron nitride layers in view of their functionality as nano-materials. Single-molecule magnetism is the focus of the fullerene research, where we apply x-ray absorption and a subKelvin superconducting quantum interference device. In the activity of the 2D materials, we grow the highest quality boron nitride on substrates up to the four-inch wafer scale with chemical vapor deposition, subsequent exfoliation, and implementation in devices. At UZH Irchel, we use a dedicated clean room, optical microscopy, inkjet printing, and surface science tools such as low-energy electron diffraction, photoemission, and scanning tunneling microscopy for these purposes. At the Swiss Light Source, we performed photoemission and x-ray absorption spectroscopy experiments.
Lifting the zero field degeneracy of 4f ion pairs
The single molecule magnetism in endohedral fullerenes with dilanthanides like Tb2 or Dy2 displays a low energy excitation that can not be understood with a pseudospin model without hybridisation. The pseudospins depict the total angular momentum states ± Jz that impose for the dilanthanides four states | ↑↑i=|1i, | ↓↑i=|2i, | ↓↓i=|1̄i and | ↑↓i=| 2̄i. In zero field the ferromagnetic pairs |1i and |1̄i are degenerate, as well as the two antiferromagnetic pairs |2i and |2̄i. With an ansatz that considers quantum tunneling of the magnetization between the four pseudospin states a tunneling rate can be determined from the Arrhenius barrier in the magneization lifetimes at sub-Kelvin temperatures. The empirical fact that the approach to thermal equilibrium contains ground state information implies that thermal fluctuations exceeding a given energy-splitting of states accelerate the equilibration. Stability of single layer h-BN on metals With an Importantly, the lifting of the zero-field degeneracy of the ground state should enable pseudospin control and manipulation. The project was funded by the Swiss National Science Foundation.
- Figure: Endohedral Tb2 ScN@C80 . The left panel shows the molecule with the two magnetically interacting Tb 4f 8 electron systems (red tori). Center experimental zero field magnetisation lifetimes (A. Kostanyan et al., Phys. Rev. B (2020)). Two Arrhenius processes are indicated. While I I with a barrier of 10.5 K corresponds to the energy difference in a ferromagnetic or an antiferromagnetic ground state, the low energy excitation I has a barrier of 1 K and is proposed to be due to the lifting of the degeneracy by hybridisation of the four pseudospin states shown on the right. | 1i (yellow) and |1̄i (blue) are degenerate. Though, they hybridise with |2i (red) and |2̄i (green), which yields a non-degenerate, non-magnetic ground state. The panel shows the four states and their connections via single pseudospin flips in Hilbert space. The step arrows indicate the quantum tunneling of the magnetisation, and the different energies of the pure states. The central logo is borrowed from the 2025 unesco International Year of Quantum Science and Technology and stands here for the mixing of the four pseudospin states.
Highlighted Publications:
- Monolayer calibration of endofullerenes with x-ray absorption from implanted keV ion doses
W.C. Lee, L. Yu, Y. Zhang, A. A. Popov, C. Coletti, B.Delley, J. Oscarsson, M. W. Ochapski, Z. M. Gebeyehu, L. Martini, M. Muntwiler, D. Primetzhofer, and T. Greber, J. Vac. Sci. Technol. A 42, 023406 (2024) - Quantum Tunneling of the Magnetization in Systems with Anisotropic 4f Ion Pairs: Rates from Low-Temperature Zero-Field Relaxation
T. Greber, ACS Omega, 9, 37183 (2024) - Benchmarking the integration of hexagonal boron nitride crystals and thin films into graphene-based van der Waals heterostructures
T. Ouaj et al., 2D Mater. 12, 015017 (2025)
Prof. Marc Janoschek - Correlated Quantum Matter
Our research is centered on genuine quantum phenomena in bulk materials that arise due to collective electronic behavior. These electronic correlations strongly couple spin, charge and lattice degrees of freedom resulting in emergent and rich low-energy physics. We study materials in which such collective quantum phenomena at the atomic-scale are borne out in exotic and functional macroscopic properties. We tune the underlying quantum interactions via external control parameters (pressure, field, strain, crystal chemistry) to understand the properties of quantum materials. For this purpose, we probe quantum matter with state-of-the-art large-scale neutron, photon and muon experiments.
A novel two-ladder quantum magnet
Low-dimensional quantum magnets are a versatile materials platform for studying the emergent many-body physics and intrinsically collective excitations that even arise in systems limited to only a few short-range interactions. Further, the availability of powerful analytical and numerical techniques to study these relatively simple effective spin Hamiltonians allows for the quantitative calculation of the collective excitations and resulting exotic properties. In cases where a real materials are approximate realization of the corresponding Hamiltonian, said material can serve as quantum simulator for predicting and studying exotic many-body quantum effects. Establishing the low-temperature structure and spin Hamiltonian of candidate materials is therefore crucial to explaining their magnetic properties, including unconventional quantum phases, phase transitions, and excited states.
An example for a well-studied model Hamiltonian is the S = 1/2 quantum spin ladder, which is described by only two short-range isotropic Heisenberg exchange interactions, Jleg for the ladder legs and Jrung for the rungs. In turn, the entire model is governed by a single ratio, α = Jleg /Jrung . Quantum spin ladders bridge the gap between one and two dimensions and have complex excitation spectra, which depend on α. In zero magnetic field the excitations have a spin gap for any finite α and vary from a single triplon branch in strong-rung ladders (α < 1/2) to weakly confined spinons in the spin-chain limit (α >> 1). Arguably the most interesting properties appear in a magnetic field sufficiently strong to close the gap, where the system becomes a spin Tomonaga-Luttinger liquid (TLL), a theoretical model describing interacting fermions in one dimension. However, also materials in the strong-leg regime with α > 1 are of interest due to the delocalized and spinonic character of their excitations. Notably, to date (C7 H10 N2 )2 CuBr4 (DIMPY) is the only clean, strong-leg ladder compound to be studied in detail.
We have performed an extensive investigation of the metal–organic coordination compound (C5 H9 NH3 )2 CuBr4(Cu-CPA), which upon its discovery was suggested as a candidate two-leg quantum spin ladder in the strong-leg coupling regime. Employing magnetization, specific heat as well as X-ray and neutron diffraction measurements, we show that Cu-CPA has a previously unreported low-temperature structure with a monoclinic unit cell that gives rise to two inequivalent spin ladder motives. We further confirm the absence of long-range magnetic order down to 30 mK. Our study identifies Cu-CPA as two-ladder model and as a candidate material to study collective magnetic excitations in the strong-leg regime. Cu-CPA may provide the interesting opportunity to study the interaction of excitations on two distinct ladders.
- Zoom (PNG, 122 KB)
- Figure: Schematic representation of the interaction parameters expected on the basis of the inequivalent atomic pathways in the two ladders of Cu-CPA. The four inequivalent leg interactions, Jleg,1a , Jleg,2a , Jleg,1b , and Jleg,2b , are shown as green, solid lines. The two inequivalent rung interactions, Jrung,a and Jrung,b , are shown respectively as dashed red and orange lines. The diagonal interactions, Jdiag,a and Jdiag,b are denoted respectively as purple and blue dotted lines. A possible small interladder interaction is not represented.
Highlighted Publications:
- (C5 H9 NH3 )2 CuBr4 : a metal-organic two-ladder quantum magnet,
J. Philippe, et al., Phys. Rev. B 110, 094101 (2024)
Prof. Fabian Natterer - Quantum Matter
Our research group focuses on low-dimensional quantum materials and quantum sensing. We explore how matter receives her properties from the interaction between individual atoms and molecules using atomically resolved scanning probe microscopy (SPM). By subjecting matter to extreme conditions - such as cryogenic temperatures, exposure to magnetic fields, or doping – we find control knobs that fine-tune a material’s property. Our investigations include measurements of the electronic structure using SPM enabled electron spin resonance, pump-probe spectroscopy, and fast quasiparticle interference imaging.
Electronic Structure Mapping of 2D Materials
We infer the band structure of two dimensional quantum materials using fast quasiparticle interference imaging (QPI). QPI works by measuring the point spectroscopy (local density of states, LDOS) at every topographic location. To speed up this traditionally slow technique, we utilize compressed sensing and parallel spectroscopy. While the former enables the measurement of fewer locations, the latter speeds up the LDOS mapping. The dynamical nature of parallel spectroscopy helped us introduce and novel measurement concept in which we actively subtract large current amplitudes before they reach the preamplifier and that would otherwise have led to saturation. HDR-STM allows us to measure spectroscopy against a vastly varying background with improved resolution at small currents that will serve to study the electron-phonon coupling in correlated quantum materials.
Atomically resolved Josephson Junction STM
We implement a Josephson junction STM (JJ-STM) to spatially map the properties of superconductors. Every Josephson junction consists of two weakly interacting superconductors, here represented by a superconducting sample and tip that are separated by a vacuum barrier. Accordingly, we grow ultrathin superconducting Nb layers and use a superconducting tip made from Nb wires. The JJ acts as the central component of a charge qubit that can be scanned along the surface and is used to atomically map sources of decoherence. Identification of these single atom impurities is crucial to futher enhance the coherence time of superconducting qubits which is key to quantum computers finally becoming a reality.
- Zoom (PNG, 200 KB)
- Figure: High-dynamic range scanning tunneling microscopy. Using an AC excitation voltage instead of DC, we compress the dynamical range of the STM, allowing us to perform tunneling spectroscopy simultaneously for large and small currents at the highest gains without preamplifier saturation. This works because the cosinusoidal excitation creates harmonics at integer multiples of the excitation frequency that can be individually suppressed by adding a cancelling displacement current through the compensating capacitor[2].
Highlighted Publications:
-
Clip-on lens for scanning tunneling luminescence microscopy,
A. Cahlík, C. Müller, F. Natterer, MethodsX, 13:102828 (2024) -
High dynamic range scanning tunneling microscopy,
A. Karić, C. Marques., B. Zengin, F. Natterer, MethodsX, 13:1028257 (2024)
Prof. Johanna Nordlander - Oxide Materials
Our work seeks to create quantum materials by design through thin film epitaxy. The thin film geometry offers a unique opportunity to explore fundamental thickness limits, dimensional cross-over effects and stabilization of novel metastable phases. Our focus is on complex oxides, where high flexibility in chemical composition and strong electronic correlations lead to rich phase diagrams that may host exotic phases of quantum matter such as high-temperature superconductors, multiferroics, spin liquids and topological phases. We aim to engineer such phases in thin films by careful design of lattice geometry to set or break symmetries at the nanoscale that in turn control the macroscopic properties of the system.
Novel functionalities in epitaxial oxides
Our group started at the University of Zurich in October 2024 and is setting up a state-of-the-art pulsed laser deposition lab to construct nanoscale single layers and heterostructures of oxide quantum materials in a monolayer-by-monolayer approach. Current research includes the thin-film realization of quantum spin liquid (QSL) candidates and exploring novel routes to characterize its frustrated magnetic ground state at low film thicknesses. In QSL materials, strongly frustrated magnetic interactions lead to highly entangled spins without long-range order even down to the lowest temperatures. The ground state of such materials is suggested to be a proximate phase to unconventional superconductivity and may provide a materials platform for topological quantum computing. Despite these prospects, realizing a thin-film counterpart to bulk QSL candidate materials remains challenging and only few such thin film systems have been reported to date.
We have recently synthesized the first thin films of TbInO3 , belonging to a family of frustrated magnets recently suggested to host a QSL ground state. Our thin films reveal the preservation of the frustrated magnetic state without spin freezing or long-range order down to at least 1 K, where further studies regarding epitaxial strain and interfacial doping may provide new tuning knobs unique to the thin film geometry to manipulate the magnetic and electronic ground states of QSL candidate systems. We are furthermore interested in nanoscale layering of functional materials that combine different types of phase instabilities such as metal-to-insulator transitions and exotic magnetic or ferroelectric phases, where interfacial coupling, strain, and proximity effects may lead to the emergence of new physics in these materials systems. To this end, we are collaborating with the Paul Drude Institute in Germany, to leverage the unique capabilities of in-situ X-ray diffraction during oxide molecular beam epitaxy located at BESSY II to design such heterostructures with monolayer precision.
- Zoom (PNG, 383 KB)
- Figure: Post-deposition surface reconstruction and phase transformation of epitaxial vanadium oxide thin films on sapphire in the presence of oxygen plasma as recorded by in-situ reflection high energy electron diffraction (RHEED).
Highlighted Publications:
- Defect Engineering in Epitaxial Thin Films of the Pyrochlore Frustrated Magnet Tb2 Ti2 O7
M. A. Anderson et al., Chem. Mater. 36, 2325 (2024) - Combined Electrostatic and Strain Engineering of BiFeO3 Thin Films at the Morphotropic Phase Boundary J. Nordlander et al., Adv. Electron. Mater. 10, 2400185 (2024)
Prof. Andreas Schilling - Phase Transitions, Materials and Applications
We are interested in selected topics in materials research, spanning the entire spectrum from searching new materials, their characterization, and corresponding applications. We have been particularly active in superconductivity, magnetism and thermodynamics. Our laboratory is equipped with modern furnaces for material synthesis, 4He/3He cryostats and a dilution cryostat, all with superconducting magnets. We are structuring thin superconducting films at the FIRST Center for Micro- and Nanoscience at ETHZ and are using them both for basic research and applications. Corresponding nanostructures may serve as ultrafast single-photon detectors in the infrared, visible and Xray range.
Superconducting ultra-thin MgB2 films
Ultra-fast single-photon detectors with high current density and operating temperature can benefit space and ground applications, including quantum optical communication systems with lightweight cryogenics for space crafts and medical use. We have recently introduced [1] magnesium diboride (MgB2 ) thin-film superconducting microwires capable of single-photon detection at 1.55 μm optical wavelength. We used helium ions [2] to alter the properties of MgB2 , resulting in microwire-based detectors exhibiting single-photon sensitivity across a broad temperature range of up to 20 K, and detection-efficiency saturation for 1 μm wide microwires at 3.7 K. The linearity of the detection rate vs. incident power was preserved up to at least 100 Mcps. Despite the large active area of up to 400x400 μm2 , the reset time was found to be as low as about 1 ns. This research provides possibilities for increasing the operating temperature limit and maximum single-pixel count rate, expanding the detector area, and raises fundamental questions about the mechanisms of single-photon detection in high-critical-temperature superconductors.
Superconducting two-dimensional (2D) NbSe2
The rapid development of infrared spectroscopy, observational astronomy, and scanning near-field microscopy has been enabled by the emergence of sensitive mid- and far-infrared photodetectors. Superconducting hot-electron bolometers (HEBs), known for their exceptional signal-to-noise ratio and fast photoresponse, play a crucial role in these applications. While superconducting HEBs are traditionally crafted from sputtered thin films such as NbN, the potential of layered van der Waals (vdW) superconductors is untapped at THz frequencies. Recently, we demonstrated [3] superconducting HEBs made from few-layer NbSe2 microwires. By improving the interface between NbSe2 and metal leads, we overcome impedance mismatch with RF readout, enabling large responsivity THz detection (0.13 to 2.5 THz) with a minimal noise equivalent power of 7 pW/Hz and nanosecond-range response time. Our work highlights NbSe2 as a promising platform for HEB technology and presents a reliable vdW assembly protocol for custom bolometer production.
- Zoom (PNG, 383 KB)
- Figure: Scanning-electron microscopy (SEM) image of a 1 μm-wide meander-shaped microwire device.
Highlighted Publications:
- Single-photon detection using large-scale high-temperature MgB2 sensors at 20 K.,
I. Charaev et al., Nat. Commun. 15 (2024) 3973. - Effects of Helium Ion Exposure on the Single-Photon Sensitivity of MgB2 and NbN Detectors,
E. Batson, et al., IEEE Trans. Appl. Supercond. 34 (2024) - Fundamental limits of few-layer NbSe2 microbolometers at terahertz frequencies,
K. Shein et al., Nano Lett. 24 (2024) 2282.
PD. Tatiana Latychevskaia (PSI) - Coherent Diffraction Imaging
Our research is focused on developing lens-less coherent imaging techniques for high-resolution and three-dimensional imaging of nano-scaled objects, two- dimensional materials (graphene, hexagonal boron nitride, transition metal dichalcogenides, etc.) and macromolecules. In coherent diffraction imaging (CDI) and holography techniques, the intensity of the wave diffracted by the sample is acquired by a detector in the far field, and the phase distribution of the diffracted wave together with the sample structure is then reconstructed by applying numerical methods. Employing short-wavelength radiation, such as electron or X-ray waves, in lens-less imaging techniques allows for imaging at atomic resolution.
Au nanoparticles on graphene imaged by convergent beam electron diffraction (CBED)
CBED on two-dimensional materials allows simultaneous recording of the real-space image (tens of nanometers in size) and diffraction pattern of the same region in the sample in one single-shot intensity measurement. We employed time-series CBED to visualize the dynamics of single Au nanoparticles deposited on graphene [1]. The real-space image of the probed region, with the amount, size, and positions of single Au nanoparticles, is directly observed in the zero-order CBED disk, while the atomic arrangement of the Au nanoparticles is available from the intensity distributions in the higher-order CBED disks (Fig. 1). It was observed that each Au nanoparticle provides its own CBED diffraction pattern superimposed with the CBED pattern of the supporting graphene. The CBED patterns of graphene and Au nanoparticles show independent behavior; thus changes in both the support and the nanoparticles could be separated. Combining the real- and diffraction-space information allowed reconstruction of
the dynamic behavior of the nanoparticle – rolling back and forth on the graphene surface. For a 5 nm in diameter Au nanoparticle, the energy of a lateral movement by 1 nm in 1 ns is calculated to be 3.942 meV, and the energy of a rotation by 5 degrees in 1 ns is 0.075 meV, giving a high probability of these movements at room temperature exp(− E/kBT) = 0.858 and 0.997, respectively; kBT = 25.6 meV. Thus, lateral and rotational movements of Au nanoparticles are highly probable at room temperature even without additional energy deposited by the electron beam. From the time-series CBED patterns, the movement of a single Au nanoparticle with rotation up to 4° was recorded. We also observed facet diffraction lines - intense bright lines formed between the CBED disks of the Au nanoparticle, which we explain by diffraction at the Au nanoparticle’s facets.
- Zoom (PNG, 522 KB)
- Figure: Convergent beam electron diffraction (CBED) of Au nanoparticles on graphene. (a) Experimental arrangement. (b) Experimental CBED pattern of graphene with one Au nanoparticle; ∆ f = −2 μm and convergence semi-angle α = 9.8 mrad; the diameter of the probed region is D ≈ 40. The red arrow indicate the CBED disks of the Au nanoparticles. The yellow arrow indicate the shadow image of the Au nanoparticle in the zero-order CBED disk. (c) High-angle annular dark-field (HAADF) images of Au nanoparticles deposited on graphene: (i) round-shaped, (ii) icosahedron or cuboctahedron, (iii) and (iv) mixed-state Au nanoparticles.
Highlighted Publications:
-
Dynamics of single Au nanoparticles on graphene simultaneously in real- and diffraction space by time-series convergent beam electron diffraction,
S. Mustafi et al, Micron 194, 103814 (2025), https://doi.org/10.1016/j.micron.2025.103814
Table of contents
- Prof. Tomáš Bzdušek - Theory of topological matter
- Prof. Titus Neupert - Condensed matter theory
- Prof. Johan Chang - Superconductivity and Magnetism
- Prof. Thomas Greber - Low dimensional systems
- Prof. Marc Janoschek - Correlated Quantum Matter
- Prof. Fabian Natterer - Quantum Matter
- Prof. Johanna Nordlander - Oxide Materials
- Prof. Andreas Schilling - Phase Transitions, Materials and Applications
- PD. Tatiana Latychevskaia (PSI) - Coherent Diffraction Imaging