Particle Physics
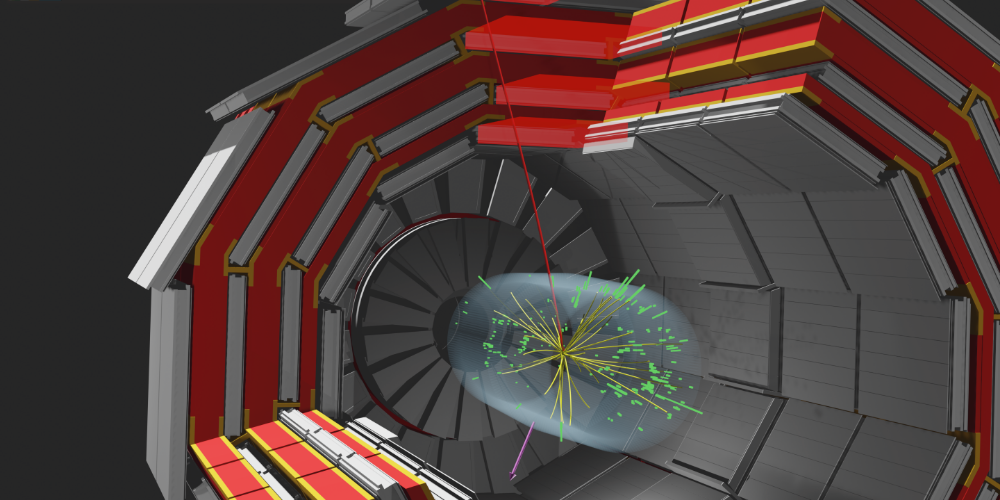
Table of contents
- Prof. Andreas Crivellin - Flavour beyond the Standard Model
- Prof. Thomas Gehrmann - Precision Calculations
- Prof. Massimiliano Grazzini - Standard Model and Higgs Physics at Colliders
- Prof. Gino Isidori - Beyond the Standard Model
- Prof. Stefano Pozzorini - Automated Simulations for high-energy colliders
- Prof. Adrian Signer - High-intensity low-energy particle physics
- Prof. Peter Stoffer - Effective Field Theories at the Precision Frontier
- Prof. Max Zoller - Precision Calculations
- Prof. Lea Caminada, Prof, Florencia Canelli, Prof. Ben Kilminster – CMS Experiment
- Profs. Lea Caminada, Florencia Canelli, Ben Kilminster - Collider detector development
- Prof. Nicola Serra, Prof. Olaf Steinkamp - LHCb Experiment
- Profs. Lea Caminada, Nicola Serra, Olaf Steinkamp - The Mu3e experiment
- Prof. Nicola Serra - SHiP Experiment
- Prof. Florencia Canelli - Future Circular Collider (FCC)
Prof. Andreas Crivellin - Flavour beyond the Standard Model
The Standard Model (SM) of particle physics describes Nature’s fundamental constituents and interactions. Matter consists of quarks and leptons (fermions) which interact via the exchange of force particles (gauge bosons). The SM has been tested with high accuracy, both in high-energy searches at the Large Hadron Collider (LHC) at CERN and in low-energy precision experiments. However, it is well known that it cannot be the ultimate theory of nature since it fails to explain observations like Dark Matter, Dark Energy, neutrino masses or the presence of more matter than anti-matter in the Universe. The goal of our research is to construct and study models of physics beyond the SM both regarding their signatures in low-energy precision experiments and direct searches at the Large Hadron Collider (LHC).
Indications for new Higgs bosons at the Large Hadron Collider
The indications for a new Higgs boson with a mass around 152 GeV in the di-photon channel, produced in association with other signatures, have further been substantiated. Our updated fit finds a significance of ≈ 4σ for an SU (2) L triplet Higgs [1] and even slightly more can be achieved in a two-Higgs-doublet model [2]. Interestingly, these indications for new scalar bosons might be connected to charge-party violation in low-energy observables such as the electron, neutron and proton electric dipole moments [3]. If confirmed, these excesses would provide a strong physics case for future colliders [4].Direct and Indirect Hints for Physics Beyond the Standard Model.
Significant tensions with the SM predictions were observed in many processes involving multiple leptons and missing energy, (possibly in conjunction with bottom quarks) at the LHC [1]. In particular, the differential top quark distributions (pp → tt → WWbb → eμbb) measured by ATLAS indicate the presence of new Higgs bosons (>5sigma) [2]. These new scalars can also be searched for at the LHC in di-photon final states. Here, we showed that a Higgs triplet can explain the excesses in γγ+X, where X can be missing energy, leptons and (bottom) quarks [3] (see Figure). In Ref. [4] we proposed a complete model which can account for the differential top quark distributions as well as for the di-photon excesses at the same time. This model predicts more new Higgs bosons which can be discovered with LHC run-3 data.
Figure: Statistical combination of the relevant di-photon channels in the triplet model. Note that a non-zero branching ratio is preferred at 152 GeV be ≈ 4σ.
Highlighted Publications:
- 1. Growing Evidence for a Higgs Triplet,
A. Crivellin, S. Ashanujjaman, S. Banik, G. Coloretti, S. P. Maharathy and B. Mellado,
arXiv:2404.14492 [hep-ph] - Explanation of the excesses in associated di-photon production at 152 GeV in 2HDM,
S. Banik and A. Crivellin,
JHEP 10 (2024), 20doi:10.1007/JHEP10(2024)203 - Correlating A → γγ with EDMs in the 2HDM in light of the diphoton excesses at 95 GeV and 152 GeV,
S. Banik, G. Coloretti, A. Crivellin and H. E. Haber,
arXiv:2412.00523 [hep-ph] - Discovery Potential of Future Electron-Positron Colliders for a 95 GeV Scalar,
P. Sharma, A. T. Mulaudzi, K. Mosala, T. Mathaha, M. Kumar, B. Mellado, A. Crivellin, M. Titov, M. Ruan and Y. Fang,
arXiv:2407.16806 [hep-ph]
Prof. Thomas Gehrmann - Precision Calculations
Our research group focuses on precision calculations for collider observables within the Standard Model and their application in the interpretation of experimental data. We develop novel techniques and computer algebra tools that enable analytical calculations in perturbative quantum field theory and help to unravel the underlying mathematical structures. We implement our results into numerical parton-level event generator programs, which are flexible tools that allow to take proper account of the details of experimental measurements, enabling precision theory to be directly confronted with the data.
Semi-inclusive hadron production in deep inelastic scattering
Semi-inclusive hadron production processes in deep inelastic lepton-nucleon scattering (SIDIS) are important probes of the quark flavour structure of the nucleon and of the fragmentation dynamics of partons into hadrons. By considering different hadron species, it is possible to single out differ-
ent flavour combinations of incoming partons, thereby probing the detailed quark and antiquark flavour decomposition of the parton distribution functions (PDFs). This SIDIS information is largely complementary to inclusive DIS structure function measurements, which allow to determine only a single flavour combination to high accuracy. Moreover, SIDIS measurements play an important role in the determination of spin-dependent PDFs that have to rely on far fewer hadron-collider observables than ordinary (spin-averaged) PDFs. Up to now, all these studies could be performed in a self-consistent manner only up to next-to-leading order (NLO) in QCD perturbation theory due to the unavailability of corrections to the SIDIS coefficient functions at higher orders.
We computed compact analytic expressions for the next-to-next-to-leading order (NNLO) QCD corrections to the parton-level coefficient functions for unpolarized and polarized SIDIS, thereby enabling these processes to be included in precision PDF studies.
Figure: A1π+Asymmetries in polarized SIDIS at different perturbative orders compared to data from COMPASS and HERMES.
NNLO is the first order where the full set of parton-to-parton transitions contributes in SIDIS processes. The newly computed corrections lead to a considerable reduction of the theory uncertainty on the predictions, especially for unpolarized SIDIS, and yield an improved description of the experimental data. In polarized SIDIS, the NNLO effects are particularly pronounced at low x (Figure).
The future electron-ion collider (EIC), currently under construction at Brookhaven, will perform an extensive measurement program of SIDIS processes for a variety of hadron species in unpolarized and polarized collisions at high energies. Our results will allow to analyse these data in future precision
Highlighted Publications:
- Semi-Inclusive Deep-Inelastic Scattering at NNLO in QCD,
L. Bonino, T. Gehrmann and G. Stagnitto,
Phys. Rev. Lett. 132 (2024) 251901. - Polarized Semi-Inclusive Deep-Inelastic Scattering at NNLO in QCD,
L. Bonino, T. Gehrmann, M. Löchner, K. Schönwald and G. Stagnitto,
Phys. Rev. Lett. 133 (2024) 211904.
Prof. Massimiliano Grazzini - Standard Model and Higgs Physics at Colliders
Our research activity is focused on the phenomenology of particle physics at high-energy colliders. We perform accurate theoretical calculations for benchmark processes at high energy colliders and we strive to make their results fully available to the community. We develop flexible numerical tools that can be used to perform these calculations with the specific selection cuts used in the experimental analyses. Our projects span over a wide range of processes from vector-boson pair production to heavy-quark and jet production, to Higgs boson studies within and beyond the Standard Model.
Link
Precise predictions for the associated production of the Higgs boson with a top-quark pair
The associated production of a Higgs boson with a tt̄ pair is a crucial process at the LHC since it allows for a direct measurement of the top-quark Yukawa coupling. At present ATLAS and CMS measure the signal strength in this channel to an accuracy of O (20%), but at the end of the High-Luminosity phase the uncertainties are expected to go down to the O( 2% ) level, thereby requiring accurate theoretical predictions. After the first results for the inclusive cross section in next-to-next-to-leading order (NNLO) QCD presented by our group in 2022, we have extended our computation to the fully differential level. The computation is exact, except for the finite part of the two-loop virtual contribution, which, for such a 2 → 3 process with several mass scales, is still beyond current possibilities. In our calculation we estimate the missing two-loop virtual contribution using two complementary methods that yield consistent results within their respective uncertainties. The first method relies on a soft-Higgs factorisation formula that we develop up to the three-loop order. The second is based on a high-energy expansion in the small top-mass limit. The former is expected to be a reasonably good approximation in the bulk region, while the latter is expected to work proved with the inclusion of NLO EW corrections.
In the Figure we show our results for the p T spectrum of the Higgs boson at different perturbative orders. We use the binning commonly adopted by the ATLAS and CMS collaborations. Our best prediction includes NNLO QCD and NLO EW corrections. The central panel shows the impact of NNLO QCD corrections on top of the NLO result. The bands denote our estimate of the perturbative uncertainties, and are obtained from the customary scale variations after symmetrisation. The inner dashed band depicts the uncertainty due to the missing two-loop contribution. The impact of NNLO corrections is positive and ranges from 5% at low pT,H to about 12% at high p T,H . The impact of NLO EW corrections is smaller, being positive at small pT,H and negative in the high p T,H region, but needs to be included at this level of precision. The residual perturbative uncertainties on the p T,H spectrum are at the 4 − 6% level. We also see that the uncertainties from the missing two-loop contribution are at the percent level, and, therefore, significantly smaller than the residual perturbative uncertainties.
- Zoom (PNG, 59 KB)
- Figure: Differential cross section as a function of the Higgs boson transverse momentum, with the binning used by the LHC experimental collaborations.
Highlighted Publications:
- Precise predictions for the associated production of a W boson with a top-antitop quark pair at the LHC,
L. Buonocore et al., arXiv:2306.16311,
Phys.Rev.Lett. 131 (2023) 23, 231901.
Prof. Gino Isidori - Beyond the Standard Model
The Standard Model of fundamental interactions describes the nature of the basic constituents of matter, the so-called quarks and leptons, and the forces through which they interact. This theory is very successful in laboratory experiments over a wide range of energies. How ever, it fails in explaining cosmological phenomena such as dark matter and dark energy. It also leaves unanswered basic questions, such as why we observe three almost identical replicas of quarks and leptons, which differ only in their mass. Finally, it gives rise to conceptual problems when extrapolated to very high energies, where quantum effects in gravitational interactions become relevant. The goal of our research activity is to formulate extensions of this theory that can solve its open problems, identifying way to test the new hypotheses about fundamental interactions in future experiments.
Probing new interactions via flavour-changing transitions
One of the key predictions of the Standard Model (SM) is that quarks and leptons do appear in three replicas (denoted generations, or flavours) that behave exactly in the same manner under the known microscopic forces, and differ only in their mass (or better their interaction with the Higgs field). Why we have three almost identical replica of quarks and leptons, and which is the origin of their different interactions with the Higgs field is one of the big open questions in particle physics. The peculiar structure of quark and lepton masses, which exhibits a strongly hierarchical pattern, is very suggestive of some underlying new dynamics that we have not identified yet. The main goal of our research activity in the last few years is trying to understand the nature of this dynamics.
To achieve this main goal, we proceed along three complementary research directions:
1) we build explicit extensions of the SM that can explain the observed pattern of quark and
lepton masses, possibly addressing also other short comings of the SM, in particular the instability of the Higgs sector;
2) we investigate the consistency of the new hypothesized interactions with current data, particularly on rare flavour-changing transitions;
3) we perform detailed predictions, according to the new hypotheses, in view of future experiments.
Over the past year, we have worked mainly along the first and second directions. First, we have constructed an extension of SM where the Higgs is a composite state, and the gauge interactions are not universal in flavor. By doing so, we have been able to address both the instability of the Higgs sector and explain in a natural way (without tuning the parameters), why third-generation fermions are heavier. Second, we have shown how rare decays into neutrino pairs (B → Kνν and K → πνν) can be uses to test the hypothesis of flavour non-universal interactions and compared the predictions of our model with recent data on these rare decays by the Belle-II and NA62 experiments.
Figure: Correlation between K → πνν and B → Kνν decay rates, normalized to the SM, in models with new physics couples mainly to the third generation. The red areas denote the parameter regions favoured at 1σ and 2σ from a global of all available data in a minimal version of the model. The grey bands indicate current experimental constraints; grey lines highlight near-future projections.
Over the past year, we have worked mainly along the first and second directions. On the model-building site, we have systematically analysed the hypothesis of flavour deconstruction, i.e. the idea that the apparent flavour universality of strong, weak and electromagnetic interactions is only an accidental low-energy property, resulting from an ultraviolet theory where gauge symmetries act differently on the different fermion families. Beside investigating the technical aspects of this hypothesis, we have shown that it explains well the observed pattern of quark and lepton masses and, at the same time, offers a general framework to construct models able to stabilise the Higgs sector.
Highlighted Publications:
- Minimal flavour deconstruction,
R. Barbieri and G. Isidori,
arXiv:2312.14004, JHEP 05 (2024) 033 - Flavour deconstructing the composite Higgs,
J. Davighi, G. Isidori and M. Pesut,
arXiv:2407.10950, JHEP 01 (2025) 041 - Probing third-generation New Physics with K → πνν
and B → Kνν, L. Allwicher et al.,
arXiv:2410.21444, Phys. Lett. B 861 (2025) 139295
Prof. Stefano Pozzorini - Automated Simulations for high-energy colliders
Our research deals with the development of automated methods for the simulation of scattering processes in quantum-field theory. The OPEN LOOPS algorithm, developed in our group, is one of the most widely used programs for the calculation of scattering amplitudes at the LHC. This tool is applicable to arbitrary collider processes up to high particle multiplicity and can account for the full spectrum of first-order quantum effects induced by strong and electroweak interactions.
Currently, new automated methods for second-order quantum effects are under development. Our phenomenological interests include topics like the strong and electroweak interactions of heavy particles at the TeV scale, or theoretical challenges related to the extraction of rare Higgs-boson and dark-matter signals in background-dominated environments. Since recently, as highlighted below, our group is also active in flavour physics studies driven by Dr. Florian Herren.
Precision simulation of top-quark pair production and decay
Semi-leptonic B-decays with multi-hadron final states
Hadrons that involve bottom quarks (b) are known as B-hadrons. Their semi-leptonic decays, which lead to final states involving hadrons and leptons, are controlled by the CKM matrix — a building block of the Standard Model (SM) of particle physics that encodes all quark-mixing effects and is very sensitive to new physics. For this reason the precise determinations of the CKM matrix elements is the object of a rich experimental and theoretical program.
The LHCb and Belle experiments are currently investigating a variety of semi-leptonic B-decays that are sensitive to the CKM matrix elements V cb and V ub . The extraction of such matrix elements from data requires an accurate theoretical understanding of the non-perturbative strong interactions that govern quarks and hadrons. For B-decays into a single hadron plus a lepton (l) and a neutrino (ν), such as B → π lν, this problem is well understood. In practice, strong interactions are embodied into form factors, F (q 2 ), which depend on the virtuality q2 of the lν system and can be parametrised in terms of a few constants that can be fitted to experimental data and numerical lattice QCD calculations.
Recently we have established the first general theoretical framework for B-decays with two hadrons in the final state [1]. Such decays are abundant and can play an important role both as signals and backgrounds. In the presence of two final-state hadrons, strong-interaction effects become highly non-trivial. The required form factors depend not only on q2 , but also on various angles and on the di-hadron invariant mass M, whose distribution reflects all resonances and non-resonant states that mediate the production of the di-hadron system. This problem was solved in a general and model-independent way by exploiting partial-wave decompositions together with dispersion relations that connect the hadronic line shape to elastic two-hadron scattering phases, which can be extracted from data or lattice QCD calculations.
As a first application we provided a consistent description of B → Dπ lν decays, a major background to |V cb | determinations in B → D l ν. The Belle experiment had reported sizeable contributions to the D–π line shape that could not be reconciled with known resonances, while our new method led to a consistent explanation of the data in terms of well-defined resonances, one of which lies below threshold (see Figure).
A very recent application of our approach to multi-hadron backgrounds to B → ρlν is being incorporated in an LHCb analysis, which will likely yield the most precise |V ub | determination in this channel. In general, our new method is applicable to a broad range of B- and D-meson decays as well as neutrino-scattering processes with multi-hadron final states.
-
Figure: Invariant mass spectrum of B+ → D − π + l+ ν. Our fit consistently describes Belle data in terms of a D*2 resonance around 2.45 GeV in combination with an S-wave with a non-trivial lineshape, which results from two overlapping broad resonances, and a sizeable contribution due to a D* resonance that lies below threshold.
Highlighted Publications:
- Model-independent description of B→Dπ lν decays,
E. Gustafson et al., Phys. Rev. D 110 (2024) 9
arXiv:2403.17501
Prof. Adrian Signer - High-intensity low-energy particle physics
Particle physics at low energy but high intensity provides an alternative road towards a better understanding of the fundamental constituents of matter and their interactions. There are numerous experiments ongoing that study the low-energy scattering of leptons on other leptons or protons, as well as hadron production through electron-positron collisions. Our group provides theory support for such experiments by computing higher-order corrections in Quantum Electrodynamics (QED) to scattering and decay processes and by systematically analysing the impact of experimental bounds on scenarios of physics beyond the Standard Model.
Monte Carlo study for low-energy electron-positron collisions
Low-energy cross sections in e+ e− collisions play a vital role in the determination of the non-perturbative hadronic contribution to the anomalous magnetic moment of the muon (AMM). Indeed, the dominant theoretical uncertainty is due to the imperfect knowledge of the hadronic vacuum polarisation (HVP). The latter can be determined through a dispersive approach from experimental data for e + e − → hadrons. Currently, the situation is very unclear, as various experimental data contradict each other and there is a tension between the traditional dispersive extraction of the HVP with more recent lattice-QCD computations. These issues make it impossible to reach a clear conclusion on whether or not the experimental result for the AMM is in agreement with the Standard Model or deviates from it (by up to 5 σ).
The dispersive evaluation of the HVP crucially depends on input from Monte Carlo codes for low-energy processes e+ e − → π + π − , e + e − → μ+ μ− , and e+ e− → e + e− , as well as the corresponding radiative processes with an additional energetic photon in the final state.
In order to compare and improve the various theoretical results, a renewed community effort RadioMonteCar-Low 2 was started with a WorkStop/ThinkStart event that took place at UZH in June 2023. During the following 18 months, we have led the RadioMonteCarLow 2 program with several further workshops and the writing of a report. The latter contains a detailed account of available results and required improvements, together with a critical comparison of the available Monte Carlo tools.
As an example of such a comparison, the figure shows the distribution of the invariant mass of the muon pair in the process e+ e− → μ + μ− γ at a centre-of-mass energy on √s = 1.02 GeV. Additional cuts were applied, closely related to those applied by the KLOE collaboration. The importance of various contributions and validity of approximations is studied. Furthermore, a solid foundation was laid to further improve the theoretical description of these processes. It is foreseen that the community effort will continue in the coming years.
Figure: Distribution of the invariant mass of the muon pair, Mμμ , in an scenario for e+ e− → μ+ μ− γ inspired by the KLOE experiment. The dashed BabaYaga@NLO line shows the LO+PS (parton shower) result with leptonic vacuum polarisation (VP), but no HVP contributions. The (overlapping) dashed Phokhara and McMule lines show the NLO result without any VP contributions.
Highlighted Publications:
- Radiative corrections and Monte Carlo tools for low- energy hadronic cross sections in e+ e− collisions,
R. Aliberti, et al. arXiv:2410.22882 [hep-ph]
Prof. Peter Stoffer - Effective Field Theories at the Precision Frontier
The research of our group is focused on indirect searches for physics beyond the Standard Model and the theoretical challenges at the precision frontier: these concern the model-independent description of non-perturbative effects due to the strong interaction at low energies as well as higher-order perturbative effects that can be described within effective field theories.
Our current research activity is mainly motivated by experimental progress at the low-energy precision frontier, such as searches for CP- or lepton-flavor-violating observables and the improved measurement of the muon anomalous magnetic moment.
Despite its success, the Standard Model (SM) of particle physics fails to explain certain observations, such as the baryon asymmetry in the universe, dark matter, or neutrino masses. Our group is interested in indirect searches for physics beyond the SM, conducted in low-energy experiments at very high precision. These observables pose interesting theoretical challenges concerning the model-independent description of effects beyond the SM, as well as non-perturbative effects due to the strong nuclear force.
Low-energy effective field theory below the weak scale (LEFT)
The indirect low-energy effects of heavy physics beyond the SM can be described by effective field theories, in particular the LEFT for observables well below the electroweak scale. In order to establish a robust connection between observables at low and high energies, we are working out the renormalization of the LEFT at next-to-leading-log accuracy in the ’t Hooft–Veltman scheme, which is the only scheme proven to be fully algebraically consistent. To address the computational challenges in this scheme, we are developing tools based on state-of-the-art computer algebra. Recently, we worked out the entire evanescent sector of the theory required for next-to-leading-log accuracy and we published the first part of the two-loop renormalization-group equations. At the hadronic scale, we are working out the complete matching of the LEFT to the gradient-flow scheme. This will enable the use of improved input from lattice QCD for hadronic matrix elements that are relevant, e.g., for the neutron electric dipole moment searched for in the n2EDM experiment at PSI.
Figure: Comparison of our result for aμHLbL (gray band) to previous phenomenological and lattice-QCD evaluations (from Ref. [2]).
Anomalous magnetic moment of the muon
The theoretical prediction of the anomalous magnetic moment of the muon is currently affected by puzzling discrepancies between lattice-QCD evaluations of hadronic vacuum polarization and hadronic cross-section measurements, but also among different experiments providing this input. We are working on dispersive approaches, in order to scrutinize these discrepancies and arrive at a consolidated theory prediction.
Besides hadronic vacuum polarization, we recently published a complete dispersive evaluation of the hadronic light-by-light contribution that meets the precision goal for a comparison to the final measurement of the anomalous magnetic moment of the muon at Fermilab.
Highlighted Publications:
- Dispersion relations for the hadronic VVA correlator,
J. Lüdtke, M. Procura, P. Stoffer,
arXiv:2410.11946 [hep-ph], submitted to JHEP - A complete dispersive evaluation of hadronic light-by-light scattering,
M. Hoferichter, P. Stoffer, M. Zillinger,
arXiv:2412.00190 [hep-ph], to appear in PRL - Renormalization-group equations of the LEFT at two loops: dimension-five effects,
L. Naterop, P. Stoffer,
arXiv:2412.13251 [hep-ph], submitted to JHEP
Prof. Max Zoller - Precision Calculations
Our research focuses on the development and application of new methods and tools for high-precision theory predictions of scattering processes at particle colliders.
Automated numerical tools are crucial in accessing a large number of interesting processes at the LHC and other experiments in order to find small signatures of new physics. We are part of the collaboration developing the widely used OPENLOOPS tool, which provides first-order quantum corrections for any collider process. In our group new methods and algorithms are developed to calculate second-order quantum corrections at the same level of automation. Our approach combines highly efficient numerical algorithms with powerful analytical methods.
Our phenomenological interests include LHC physics as well as higher-order predictions for lepton experiments at lower energy.
Automation of NNLO calculations for collider physics
The LHC and future experiments demand high-precision predictions at next-to-next-to-leading order (NNLO) and beyond in quantum field theory for a wide range of scattering processes. In our group we develop an automated numerical tool for this purpose in the OPENLOOPS framework.
Our approach structures these highly complex calculations into three main tasks corresponding to a decomposition of scattering amplitudes into three building blocks. High-energy scattering amplitudes are expressed in terms of tensor integrals over the energies and momenta of virtual particles, the corresponding tensor coefficients, and the treatment of the effects of integral divergences. These three ingredients have different levels of generality.
First, the tensor coefficients are specific to each given process, and are computed with a highly efficient numerical algorithm, that has been developed and implemented in our group. For this task we exploit the general structure of two-loop Feynman diagrams (see figure).
Figure: General structure of a two-loop diagram factorising into chains Ci depending on loop momenta qi , and connecting vertices V a . The chains further factorise into process-independent segments. These properties are at the heart of all building blocks of the NNLO tool developed in this group.
These components of a full scattering amplitude factorise into a small set of process-independent building blocks, from which we compute the amplitude in a recursive way.
Secondly, the often large set of tensor integrals, usually shared by a whole class of processes, needs to be reduced to a small set of scalar master integrals. To this end we developed an algorithm combining numerical and analytical methods to achieve a balance of strong CPU efficiency and numerical accuracy in this part of the calculation. After first promising efficiency tests, this algorithm is currently being implemented into a new tool for the reduction of tensor integrals. While this tool can be tested and used independently, it is designed to be interfaced seamlessly into the other components of the NNLO OPENLOOPS framework, for which the goal is to be applied as a single automated tool.
Lastly, the treatment of integral divergences is achieved through subtraction terms, which depend only on the given particle model but not on the individual process. They are derived analytically and inserted into lower-order amplitudes of the given scattering process. The fully automated calculation and combination of these different contributions has been implemented in the NNLO OPENLOOPS framework. While the effects of ultraviolet origin are completely understood and the corresponding subtraction terms have been implemented, the effects of infrared divergences are part of our current research.
Highlighted Publications:
- Status of two-loop automation in OpenLoops,
N. Schär and M. F. Zoller,
PoS LL2024 (2024) 073, 2412.14856
Prof. Lea Caminada, Prof, Florencia Canelli, Prof. Ben Kilminster – CMS Experiment
The CMS (Compact Muon Solenoid) experiment at CERN explores the properties and interactions of fundamental particles, paving the way for discoveries. Its detector precisely measures particle energies and trajectories around an LHC collision point, where events recreate energy densities reminiscent of those just ten billionths of a second after the Big Bang. In 2012, CMS confirmed the Higgs field’s role in mass generation by discovering the Higgs boson—a major milestone for the Standard Model. Yet challenges such as the hierarchy problem, dark matter, and matter–antimatter asymmetry remain. In 2024, CMS collected Run 3 data from proton-proton collisions at 13.6 TeV (a run that began in 2022 and concludes in 2026) while preparing for a detector upgrade for the High-Luminosity LHC, set to start in 2030, to continue addressing fundamental questions and pushing the frontiers of particle physics.
- The CMS group at UZH excels in data analysis, tackling some of the most fundamental open questions in particle physics. In 2024, the UZH CMS group produced ten publications and has several additional manuscripts under submission, covering a wide range of results. We investigate the Higgs boson to deepen our understanding of its properties and use it as a tool to probe potential new forces and particles. Our research also explores uncharted regions of phase space in the search for new physics while precise measurements of Standard Model (SM) processes help uncover rare and intriguing phenomena.
- The production of Higgs bosons at the LHC involves several mechanisms, including gluon-gluon fusion (ggH), vector boson fusion (VBF), associated production with vector bosons (VH), top quark-associated production (ttH and tH), and bottom quark-associated production (bbH). To date, all these mechanisms except bbH have been observed. In addition, very rare production modes, such as charm-associated production (cH), are beginning to be explored.
- Zoom (PNG, 132 KB)
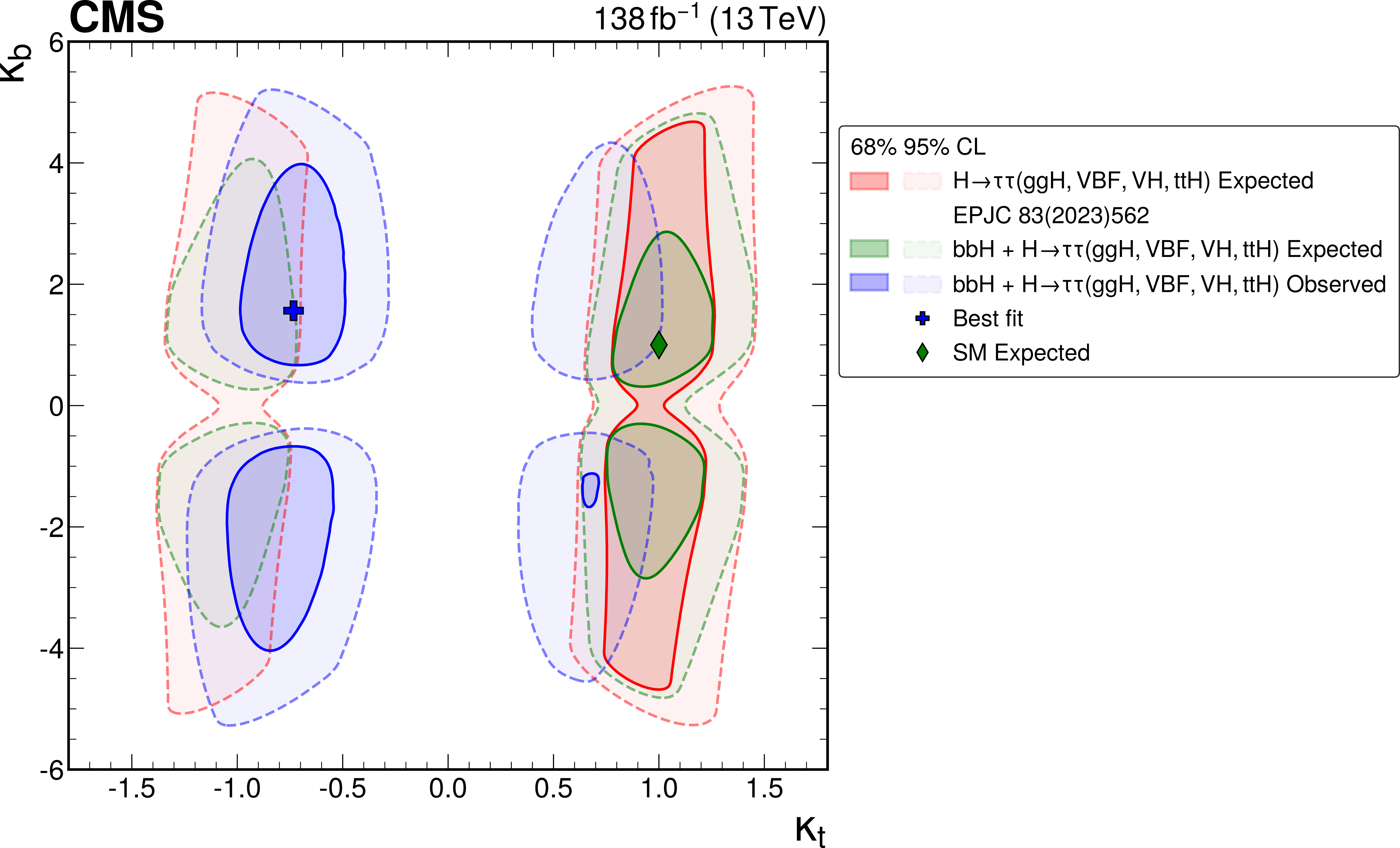
Fig. 1: Two-dimensional confidence intervals on the κ b and κ t parameters for the channels studied in the bbH search.
Although the predicted cross section for bbH is nearly equivalent to that of ttH, distinguishing bbH from background processes is more challenging due to interference with other production mechanisms, notably ggH. In our recent work, we published the first search for bbH production using the full Run 2 data, focusing on four final states: eμ, μτ h , eτ h , and τ h τ h . The observed (expected) upper limit on bbH production is found to be 3.7 (6.1) times the SM prediction at 95% confidence level [1]. The search also constrained the Higgs Yukawa couplings to bottom and top quarks in the κ-model interpretation, as shown in Fig. 1.
We have also released the first search for cH production, which targets the diphoton decay channel of the Higgs boson. This process provides a unique opportunity to probe the Higgs boson–charm quark coupling. Based on the full Run 2 data and assuming SM cross sections and branching ratios for non-cH processes, the observed (expected) upper limit on the cH signal strength is 243 (355) times the SM prediction at 95% confidence level [2].
High-energy hadron collisions require modeling nonperturbative QCD, particularly the intrinsic transverse momentum (k T ) of partons. Approaches include p T -dependent PDFs and lattice QCD. Monte Carlo generators like PYTHIA, HERWIG, and SHERPA use collinear PDFs and a tunable Gaussian for kT , compensating for shower limitations. With its clean final state, the low-p T Drell–Yan (DY) region is susceptible to k T effects. We analyze the dilepton transverse momentum in DY events over wide energy and invariant mass ranges and observe that the k T energy scaling is independent of the dilepton invariant mass. We have tuned the intrinsic k T models in PYTHIA and HERWIG to DY measurements using CMS data that extend the hard-scattering scale to 1 TeV [3].
We measured event-shape variables in low-pileup proton–proton collisions to enhance our understanding of non-perturbative QCD. Recent observations of particle correlations and strange hadron multiplicities reveal that current models – even those incorporating collective flow and rope hadronization – do not fully capture soft QCD dynamics. Moreover, the prospect of observing QCD instanton effects, which are expected to yield isotropic particle distributions, further motivates this study. We find that existing models underpredict the observed isotropy by comparing detector corrected charged-particle distributions (both inclusive and as a function of multiplicity) with simulations (Fig. 3) [4].
Figure 2: The unfolded results for transverse sphericity compared to different predictions.
We have produced the first combined effective field theory (SMEFT) interpretation of CMS data to search for indirect signs of physics beyond the SM. By simultaneously fitting seven CMS measurements – probing Higgs, electroweak vector boson, top quark, and multi-jet production – and incorporating electroweak precision observables from LEP and SLC, the study constrains 64 individual Wilson coefficients and 42 linear combinations of these coefficients. The resulting 95% confidence intervals, which translate into lower limits on the new physics scale, improve upon previous single-analysis constraints. Notably, the fit yields a 1.7% p-value for compatibility with the SM, suggesting potential deviations that warrant further investigation [5].
The tau lepton’s notoriously short lifetime of 10 −13 seconds makes its properties difficult to determine using conventional methods. In 2023, we reported the first LHC measurement of the tau lepton’s anomalous magnetic moment, (g − 2)τ , by leveraging special datasets in which Pb ions – producing dense photon fields – are collided to generate tau leptons electromagnetically. In 2024, we refined this measurement with a dataset four times larger, three additional decay modes, and a novel method that incorporates event shape analysis, resulting in a (g − 2)τ measurement that is four times more precise than our previous result [7].
Additionally, in Pb ion collisions, we first observed a process yielding two J/ψ mesons [8]. This measurement provides insight into a challenging-to-model scenario where two sets of partons interact simultaneously in a single collision. UZH scientists are leading the investigation of such double parton scattering events through detailed analyses and by organizing international workshops.
Fig. 3: In this newly considered final state, a pair of tau leptons decays into a muon, one charged particle ("prong"), and neutrinos. Using this and other kinematic distributions, the UZH group extracts the anomalous magnetic moment of the tau lepton.
Highlighted Publications:
- Search for bottom quark associated production of the standard model Higgs boson in final states with leptons in proton-proton collisions at √s = 13 TeV.
Phys. Lett. B 860 (2024) 139173. - Search for Higgs boson production in association with a charm quark in the diphoton decay channel.
HIG-23-010 (targeting JHEP). - Energy-scaling behavior of intrinsic transverse momentum parameters in Drell-Yan simulation.
GEN-22-001 (accepted by Phys. Rev. D). - Measurement of event shapes in minimum-bias events from proton-proton collisions at √s = 13 TeV.
CMS-SMP-23-008 (submitting to Phys. Rev. D). - Combined effective field theory interpretation of Higgs boson, electroweak vector boson, top quark, and multi-jet measurements. CMS-SMP-24-003 (targetting EPJC).
- Measurement of the tau g-2 factor in ultraperipheral PbPb collisions recorded by the CMS experiment.
CMS-HIN-24-011 (targeting PRL). - Observation of double J/Ψ meson production in pPb collisions at √SNN = 8.16 TeV.
Phys. Rev. D 110 (2024) 092002.
More publications at: https://www.physik.uzh.ch/r/cms
Profs. Lea Caminada, Florencia Canelli, Ben Kilminster - Collider detector development

The CMS detector includes a silicon pixel detector as the innermost part of the tracking system. The pixel detector provides 3-dimensional space points in the region closest to the interaction point that allow for high-precision tracking of charged particles and vertex reconstruction. This enables the measurement and search for particles that decay to b quarks and tau leptons, such as the Higgs boson, the top quark, and leptoquarks. Our groups are major contributors to the CMS pixel detector project. We helped build and operate the current pixel detector and are involved in the design and prototyping of a new, improved version with more tracking layers, less material, and higher data rates to be installed in 2029 for high-luminosity LHC (HL-LHC). Furthermore, we are developing and testing new pixel detector concepts for future upgrades of CMS, future accelerators and other applications.
In 2022, the UZH group replaced the innermost layer of the pixel detector in order to maintain efficient tracking during Run 3. The new layer has been successfully operated with the rest of the detector since 2022 and our groups contribute significantly to its operation and monitoring [1].
CMS will collect more than 20 times the current data set during the period of 2030 to 2041 (during HL-LHC). The UZH group together with PSI, will build an inner tracking detector for this period, that will extend the tracking coverage. This Tracker Extended Pixel detector (TEPX) will consist of a large-area disk system with more than one billion pixels [2]. At UZH, we have contributed to the module concept and we are developing the disk electronics, components of the pixel detector readout chain as well as lightweight mechanical structures and thin-walled cooling tubes to build the disk structures with minimal material. We tested pixel detector modules integrated with a prototype disk (Figure) and characterized the thermal behaviour and the performance of the novel serial powering scheme. After the successful validation we are now moving towards production of the pixel modules and detector system.
We are also developing new sensors, called Low Gain Avalanche Detectors (LGADs), which represent the optimal technology to achieve 4D tracking, combining the fine segmentation typical of silicon detectors with fast and enhanced signals to reach around 30 ps of timing resolution for minimum ionizing particles. LGADs thus open the way for precise timing together with precise positioning. Our group is characterizing different technologies within this family of silicon devices to identify the more suitable ones to work in the harsh environment of the pixel systems at LHC. For this purpose, we have been probing the timing resolution and the hit efficiency of LGAD sensors before and after irradiating them to reproduce the effect of high particle fluxes [3].
- Zoom (PNG, 439 KB)
- Figure: Prototype TEPX disk with modules mounted.
Highlighted Publications
- Development of the CMS detector for the CERN LHC Run 3,
CMS Collab., arXiv:2309.05466 - Performance and characterisation of the Phase-2 CMS Inner Tracker Endcap pixel detector,
W. Jin on behalf of the CMS tracker group,
PoS EPS-HEP2023 (2024) 578 - haracterization of timing and spacial resolution of novel TI-LGAD structures before and after irradiation,
M. Senger et al., NIMA 1039 (2022) 167030
Prof. Nicola Serra, Prof. Olaf Steinkamp - LHCb Experiment
LHCb is an experiment for precision measurements of observables in the decays of B mesons at the Large Hadron Collider (LHC) at CERN. We play a leading role in measurements with B meson decays and in measurements of electroweak gauge boson production, and have made important contributions to the LHCb detector. We contribute to an ongoing major upgrade of the detector for 2023 and are involved in studies for future upgrades of the experiment.
New LHCb measurements test behaviour of the third generation
Of the constraints on new physics, those from quark flavour physics, such as CP violation and mixing measurements, are particularly stringent with mass scale sensitivity up to 1000 TeV. The fact that the flavour sector has been consistent with the SM therefore suggests that new physics is too heavy to solve the hierarchy problem.
However, this mass scale sensitivity assumes that new physics couples equally to the three generations of fermions. If the flavour structure of new physics is non-trivial, such a hierarchical coupling to the three generations, then the largest sensitivity is in decays involving third generation fermions such as the beauty quark and τ lepton.
The decays b → cτντ are particularly interesting in this regard as they contain three third generation fermions and decay rate can be reliably predicted in the SM due to their semileptonic nature. Last year the LHCb collaboration has recently presented a new test of these decays by comparing the decay rate of transitions involving the τ lepton and the muon via so-called lepton universality ratios, R( D+ ) and R( D∗ ). This measurement was led by the group here in UZH.
The analysis measured the R( D+ ) ratio for the first time, which compared the B → Dτντ and B → Dμνμ decay rates. This particular observable is more complicated than existing LHCb measurements as the D meson is a ground state particle, meaning that there is much more background from excited states such as D∗ mesons. The τ lepton is reconstructed via the decay τ → μνμ ντ , meaning that the muonic and tauonic decays have the same final state which cancels systematic uncertainties associated with the efficiency. The main challenge is therefore to control for the background, as it cannot be easily distinguished from signal due to the presence of multiple neutrinos in the final state. The analysis is performed by fitting a three-dimensional distribution of kinematic variables which discriminate the signal from the background. Such variables include the energy of the final state lepton and the mass of the system of missing particles. Isolation criteria against additional particles is crucial to reduce the background. This criteria is extensively used to create control samples which are vital to verify that the background is under control.
The results of the new measurement is shown in the figure, along with previous results. The combination of results, shown in red, is around three standard deviations from the SM. The discrepancy from the SM hints to towards new particles at the TeV scale which preferentially couple to third generation fermions.
- Zoom (PNG, 143 KB)
- Figure: Measurements of R(D) and R(D∗ ) along with the Standard Model prediction shown in black. The single measurements of R ( D∗ ) are shown as the coloured data points whereas the joint measurements including R(D ) are shown as ellipses.
Highlighted Publications:
- All LHCb publications: lhcb.web.cern.ch/lhcb/
- Measurement of the Branching Fraction Ratios R(D+)and R(D*+) Using Muonic τ,
LHCb Collab., Phys. Rev. Lett 134, 061801
https://doi.org/10.1103/PhysRevLett.134.061801
Profs. Lea Caminada, Nicola Serra, Olaf Steinkamp - The Mu3e experiment
The mu3e experiment at PSI aims at probing the Standard Model of particle physics by searching for the decay of positively charged muons to two positrons and an electron. The observation of this decay would falsify one of the central assumptions of the Standard Model and provide unequivocal proof of "new" physics. The measurement is challenging and requires the development of novel detector techniques, which are also of significant interest for experiments at future collider facilities.
In a first phase of the experiment, the mue3 collaboration aims at exploiting existing muon beams at PSI to improve on the currently best upper limit by three orders of magnitude. The sensitivity of the experiment relies on efficient suppression of backgrounds, which necessitates precise measurements of the origins, momenta and production times of the low-energy positrons and electrons produced in decays of muons at rest. To match these requirements on measurement precision, the design of the detector incorporates a number of novel technologies. In particular, the reconstruction of the production vertices and trajectories of positrons and electrons relies on HV-MAPS, Monolithic Active Pixel Sensors produced in a commercial High-Voltage tolerant CMOS process. After an extensive R&D phase, the final version of HV-MAPS for mu3e has been produced and first detector modules have been assembled. Our group plays an important role in the assembly and testing of components for the vertex detector as well as in the development of the necessary quality assurance tools..
- Zoom (PNG, 302 KB)
- One of the first modules of the mu3e vertex detector.
.
Prof. Nicola Serra - SHiP Experiment
Our group is one of the main proponents of the SHiP (Search for Hidden Particles) experiment, which is a beam dump experiment at the SPS of CERN aiming to search for Feebly Interacting Particles (FIPs). These particles are predicted by several extensions of the Standard Model explaining Dark Matter.
Full steam ahead for the SHiP experiment
A new experiment SHiP has just been given the green light by CERN to proceed. The experiment is designed to search for so-called "hidden particles", named as such because they barely interact with ordinary matter. An example of this is the search for a heavy neutrino, which is a kind of neutral electron that could solve some of the pressing open questions such as to what is the mysterious material Dark Matter. The search for hidden particles complements the existing experiments at CERN, which instead look for signs of heavy new particles (such as what we do at the LHCb experiment here in UZH).
The SHiP experiment is laid out as shown in the Figure, with 450 GeV protons impinging on a Tungsten/Molybdenum target, followed by a hadron absorber to just leave muons and neutrinos originating from the beam. The vast majority of the muons are swept out by an active muon shield, situated just beyond the hadron absorber. Beyond is a large decay volume designed to be sensitive to the decays of feebly interacting particles. These decays will be recorded by a spectrometer located downstream of the decay volume. Now that the experiment has been approved, the next challenge will be to finalise the design, which will happen in the coming years.
Here at UZH we are particularly interested in using machine learning techniques to optimise the design and efficiently produce simulation, which is a huge challenge given the high intensity of the input beam. We are currently involved in the optimisation of the muon shield, which has the challenge of reducing the muon flux by six orders of magnitude. The challenge is to optimally design a series of six magnets in order to deflect muons (and anti-muons) with a wide range of momentum and directions. This is complicated by engineering constraints which restrict the geometry of the final detector. At UZH we are leading the optimisation of this system using machine learning, which determines the best configuration of magnets that can be feasibly build, while also taking into account the cost. The outcome of this study is crucial to the rest of the experiment, where muons form a major background in the search for hidden particles.
The group at UZH have been among the initiators of this experiment, contributing to the design, optimization, background and sensitivity studies. We hosted in the physics institute of the University of Zurich the First SHiP Workshop, where the collaboration was initially formed and the SHiP Physics Programme was discussed. Later this year, we will host the SHiP collaboration meeting again in UZH, to discuss the details of the next stage of the experiment but also to celebrate!
Figure: Experimental layout of the SHiP experiment.
Highlighted Publications
Physics Instrument Design with Reinforcement Learning,
A. R. Qasim, P. Owen, N. Serra,
https://doi.org/10.48550/arXiv.2412.10237
Prof. Florencia Canelli - Future Circular Collider (FCC)
The FCC project aims to push both the intensity and energy frontiers, seeking answers to fundamental questions about the Universe. Envisioned as the successor to the LHC, it would serve the global particle physics community well into the 21st century. Its first phase, FCC-ee, will collide e+ e− pairs in unprecedented quantities at energies ranging from about 90 to 365 GeV.
The UZH FCC group develops advanced tracking detectors and algorithms while exploring novel detector technologies to enhance and broaden the FCC-ee physics program. Over the past few years, our primary focus has been providing key insights to support the FCC Feasibility Study, slated for completion by 2025.
Last year marked an important step in our group’s efforts to explore the feasibility of the FCC. In February 2024, the midterm report of the FCC Feasibility Study was completed [1], with our group making a significant contribution through a detailed study of the IDEA vertex detector [2]. Our group advanced the development of an ultra-light vertex detector concept for FCC-ee, inspired by the ALICE ITS3 tracker upgrade. Figure 1 displays the full simulation of the design, where thin, curved, wafer-scale sensors form half-cylindrical layers around the beam pipe. This innovative configuration reduces the material budget by a factor of 3.3 compared to the current FCC-ee vertex design, enabling more precise vertex measurements and allowing the extension of the FCC-ee physics program beyond the current prospects.
- Zoom (PNG, 218 KB)
- Figure 1: Longitudinal cross-section of the four-layer ultra-light inner vertex detector concept for FCC-ee [2].
FCC-ee vertex detectors have extremely stringent requirements that can only be met by monolithic active pixel sensors (MAPS). Our group is developing MAPS using the 65 nm TPSCo process, aiming for a 3 μm single-hit resolution while achieving timing resolutions on the order of 20–500 ns and supporting high particle hit rates (approximately 200 MHz/cm2 ). In 2024, we finalized our work on the analogue pixel test structure (APTS) prototype in collaboration with ALICE collaboration [3] and further characterized the CE-65 prototype chip. In both prototypes, we demonstrated that a 3 μm resolution is achievable across various process parameters and pixel pitch combinations [3,4].
Additionally, the group finalized the development of DeepJetFCC [5], a multiclassifier neural network built on a transformer-based architecture. This flavour tagger demonstrates strong discrimination power for strange jets, as illustrated in Fig. 2. We further showed that FCC-ee could detect Z → ss̄ events in less than one second at the Z pole.
- Zoom (PNG, 196 KB)
- Figure 2: Strange tagging performance of DeepJetFCC at √s of 91.2 (Z) and 240 GeV (ZH) [5].
Highlighted Publications:
- FCC Midterm Report, B. Auchmann et al.,
doi:10.17181/mhas5-1f263 - Design, performance and future prospects of vertex detectors at the FCC-ee, PoS(ICHEP2024),
A. Ilg et al., doi:10.22323/1.476.1062 - Characterization of analogue MAPS test structures implemented in a 65 nm CMOS imaging process, NIMA,
K. Gautam et al., doi:10.1016/j.nima.2024.169896 - Characterisation of analogue MAPS fabricated in 65 nm technology for the ALICE ITS3, NIMA,
K. Gautam et al., doi:10.1016/j.nima.2024.169787 - Tagging more quark jet flavours at FCC-ee at 91 GeV with a transformer-based neural network, EPJC,
F. Canelli et al., doi:10.1140/epjc/s10052-025-13785-y
Table of contents
- Prof. Andreas Crivellin - Flavour beyond the Standard Model
- Prof. Thomas Gehrmann - Precision Calculations
- Prof. Massimiliano Grazzini - Standard Model and Higgs Physics at Colliders
- Prof. Gino Isidori - Beyond the Standard Model
- Prof. Stefano Pozzorini - Automated Simulations for high-energy colliders
- Prof. Adrian Signer - High-intensity low-energy particle physics
- Prof. Peter Stoffer - Effective Field Theories at the Precision Frontier
- Prof. Max Zoller - Precision Calculations
- Prof. Lea Caminada, Prof, Florencia Canelli, Prof. Ben Kilminster – CMS Experiment
- Profs. Lea Caminada, Florencia Canelli, Ben Kilminster - Collider detector development
- Prof. Nicola Serra, Prof. Olaf Steinkamp - LHCb Experiment
- Profs. Lea Caminada, Nicola Serra, Olaf Steinkamp - The Mu3e experiment
- Prof. Nicola Serra - SHiP Experiment
- Prof. Florencia Canelli - Future Circular Collider (FCC)